Our latest paper on a real-time reaction control system for the solar production of chemicals under fluctuating irradiance was published online today in Green Chemistry (DOI: 10.1039/c8gc00613j). Our solar-photochemistry research has always been characterized by the desire to reduce the gap between the lab and the rooftop, in other words we want to enable the use of sunlight as a free light source for many photochemical reactions in a simple and efficient way. With the Luminescent Solar Concentrator PhotoMicroreactor (LSC-PM) design we introduced a novel way to convert the polychromatic solar spectrum into a narrow band, while now we addressed the issue of light intensity fluctuations (e.g. due to passing clouds).1 Here, we will give you some inside information on our solution for this important issue within solar photochemistry.
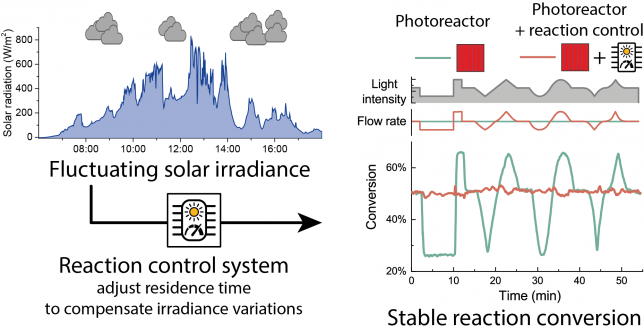
The problem with sunlight variable intensity
To the chemist inclined to perform photochemical reactions with solar light, one of the main limitations is represented by the uncontrollable fluctuations in solar irradiance. In particular, while the daily and seasonal variation can be predicted, short fluctuations introduced by passing clouds are the most difficult to address. In the past, we experienced this first-hand when we tested outdoors our reactions (e.g. see Figure2).
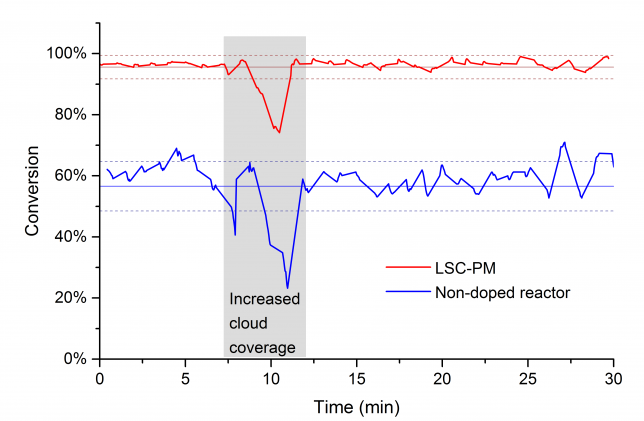
The idea
To use the words of an anonymous reviewer, “It is common knowledge that the kinetics of photoreactions are often governed by the supplied photon flux.” Using a light sensor for adjusting the irradiation time to compensate the different irradiance might, therefore, seem trivial. Alas, reality is unfortunately far from that!
For example, the spectral distribution of solar light can change significantly throughout the day. Other than the obvious red color of sunlight at sunset and sunrise, also cloud coverage, atmospheric humidity, ground albedo and other parameters can affect the wavelength distribution of solar radiation at the ground. The only reason why we could keep our system simple is that we used a Luminescent Solar Concentrator PhotoMicroreactor (LSC-PM). In an LSC-PM, the incoming solar radiation is absorbed by a fluorescent dye that re-emits the absorbed photons in a polymeric lightguide as a down converted and narrowband beam corresponding to the dye fluorescence spectrum (see Figure 3). As a consequence of the adoption of the LSC-PM design, we could neglect the variation in sunlight spectral distribution! Furthermore, by placing a light sensor in direct contact with the device edge, the light intensity measured is proportional to the photon-flux experienced by the reaction mixture flowing in the reactor channels.2
Figure 3 LSC-PM working principle. The solar photons reaching the device are either reflected (1), transmitted (2) or absorbed within the device. Most of the photons are absorbed by the dispersed dye molecules (red dot) that re-emit fluorescent photons that are waveguided to the reaction channels (5a) or to the device edge (4).Eventually, our reaction control system is simple and elegant. As in the quote often attributed to Leonardo da Vinci, we could say that simplicity, in this case, “is the ultimate sophistication”. 🙂
Merging chemistry and electronics
Being aware of the relationship between the light intensity emitted at the device edge and the photon flux in the reaction channel, we had to find a way to measure the former as an estimation for the latter. Thanks to the advancements in electronics, that is not a difficult task. After all, most of our smartphones have a little sensor to adjust the screen backlight to the ambient light conditions.
After a brief screening of different light sensors (photoresistors, phototransistor, integrated circuits based on PV cells) we decided to use a phototransistor thanks to its simplicity. By including it in a voltage divider circuit, the variation in the light intensity can be measured as a variation in the voltage drop across a resistor connected in series (see circuit in Figure 4).
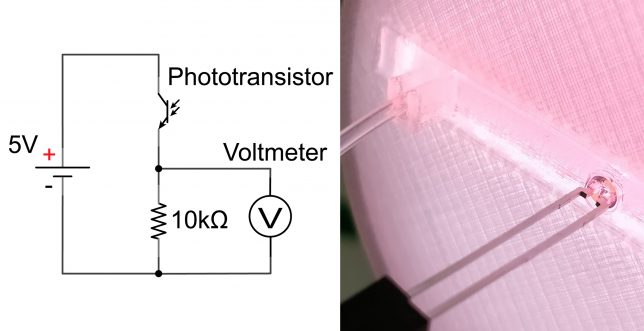
Kinetic data
With a working sensor in our hands, we needed to perform the calibration between the different light intensity levels, and the corresponding conversion at different residence time. This can be a rather laborious work but, as you might have noticed at this point, in our group we are not afraid of merging chemistry and technology. Therefore, we have developed a simple python script to control pump flow rate and LED light intensity (with a MOSFET) while measuring the reaction conversion was measured in line with a UV-VIS spectrometer. As such, this data can be obtained in fast, automated and reliable fashion.
Timing is everything
After gathering all the required data, finding the voltage/residence time relationship to keep the conversion stable was relatively easy. The first tests of the system with an artificial set of light variation were both encouraging and alarming. While the system was actually capable of adjusting the residence time to match the variation of light intensity, its reactiveness was too slow.
For a moment we feared that this might be an intrinsic limitation of our reactor (e.g. due to its elastic nature or modest volume). It turned out that the major source of variability was the delay between the light intensity reading and the adjustment of the pump flow rate. While the phototransistor responsiveness to light variation was almost instantaneous3, most of the delay was in the computer controlling the pump and the pump actually changing the flow rate. By reducing the loop time in the reaction control script to the pump response time, an update rate of less than a second was achieved. After this correction, a good stability in the reaction conversion was obtained even for large and fast variation to the external irradiance.
Arduino
Up to this point, we had been using a PC to interface with a voltmeter to read the voltage drop and to change the pump flow rate accordingly. The system was fine for lab development, but clearly, we could not propose to use it as such to control multiple reactors in a hypothetic solar mini-plant. The advantages of switching from a reaction analysis approach to monitor the reaction progress to a physical measurement that directly addresses the source of variability and that can predict the reaction outcome represented a significant conceptual difference.
We went back to the drawing board and decided to simplify the overall system with the adoption of an Arduino microcontroller. Once programmed with the kinetic investigation data, the controller would automatically send commands to the syringe pump to correct the flow rate based on the voltage measured in the light sensor. In this way, both the multimeter and the PC are replaced by an inexpensive integrated circuit. Tim particularly liked the inexpensive nature of this solution, whose total cost was about 50€. Actually, by using a generic Arduino instead of a genuine one or by including all the components in a single printed circuit board, the price of the system could be decreased to less than 10€ per piece (and even further in case of mass production). We believe that this system has potential to be applied for the reaction control of every solar photoreactor tested outdoors. To describe it with the words of another anonymous reviewer: “The potential of this to thus be completely automated will bring legitimacy to the idea of actually using solar to perform synthetic organic chemistry in practice”. And that is exactly the goal we are working towards!
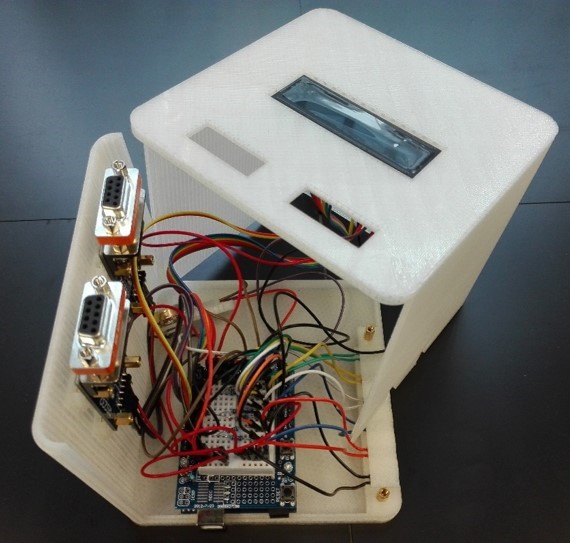
Outdoor experiment
Finally, as usual in our LSC-PM papers, we tested the reaction control system outdoors on a cloudy day, luckily those cloudy days are quite common here in Eindhoven… The system worked as planned and could handle challenging weather conditions (read: frequent and steep variations due to passing clouds). Once we got these results, we went back to the office with a big smile and finalized the paper writing process.
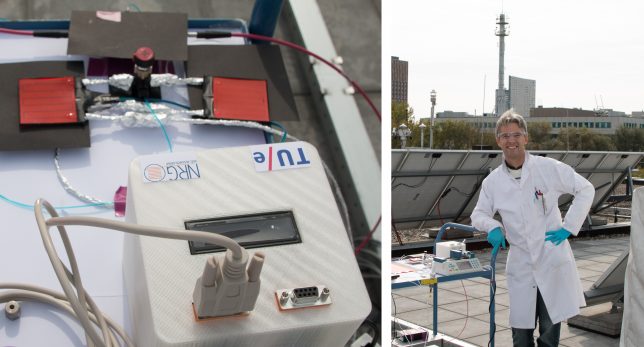
Outlook
It is undeniable that the dilute nature of solar energy will never make it suitable for the production of bulk chemicals. The volumes and margins of fine and specialty chemicals, on the other hand, constitute a real possibility for solar photochemistry. One should also realize that the use of solar energy is basically for free and abundantly available. Especially when energy prices will rise (it is just a matter of time), these technological solutions might make the difference! We believe that our paper is yet another step in our lab’s effort towards simple and more efficient solar photoreactors. Ultimately, we hope to see our technology implemented in the solar synthesis of some life-saving drugs! 🙂 🙂
Bye!
Fang, Dario, and Tim
1 To this is particularly relevant the Whitesides’ recent perspective on the future of organic chemistry: “Another possible direction for the future evolution of Organic Synthesis is, in effect, to fuse (at least in part) with Chemical Engineering, and use the techniques of that area to simplify processes […]”
2 More details on this in the Monte Carlo ray-tracing simulations that we have published last year.
3 10 µs according to manufacturer specifics